Recent posts
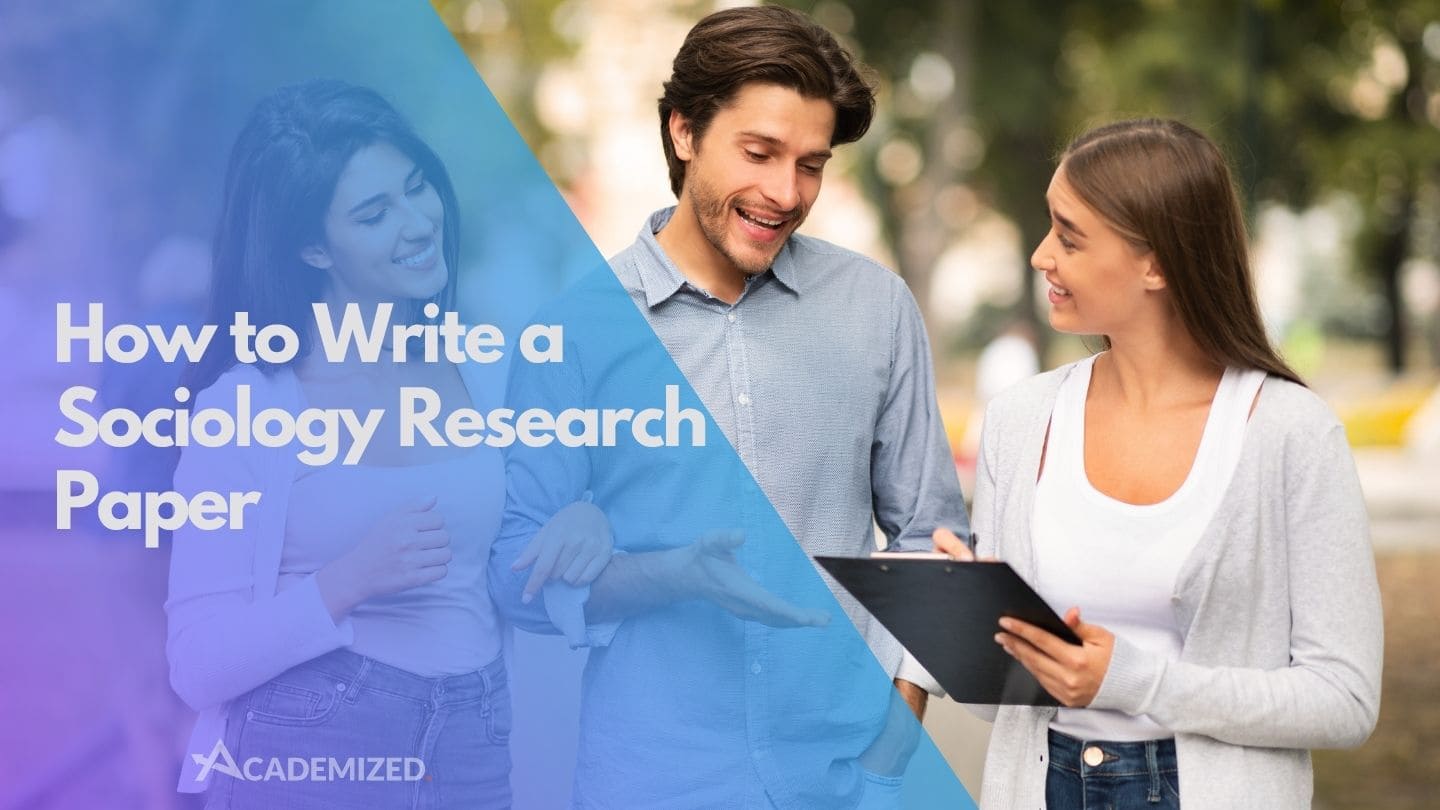
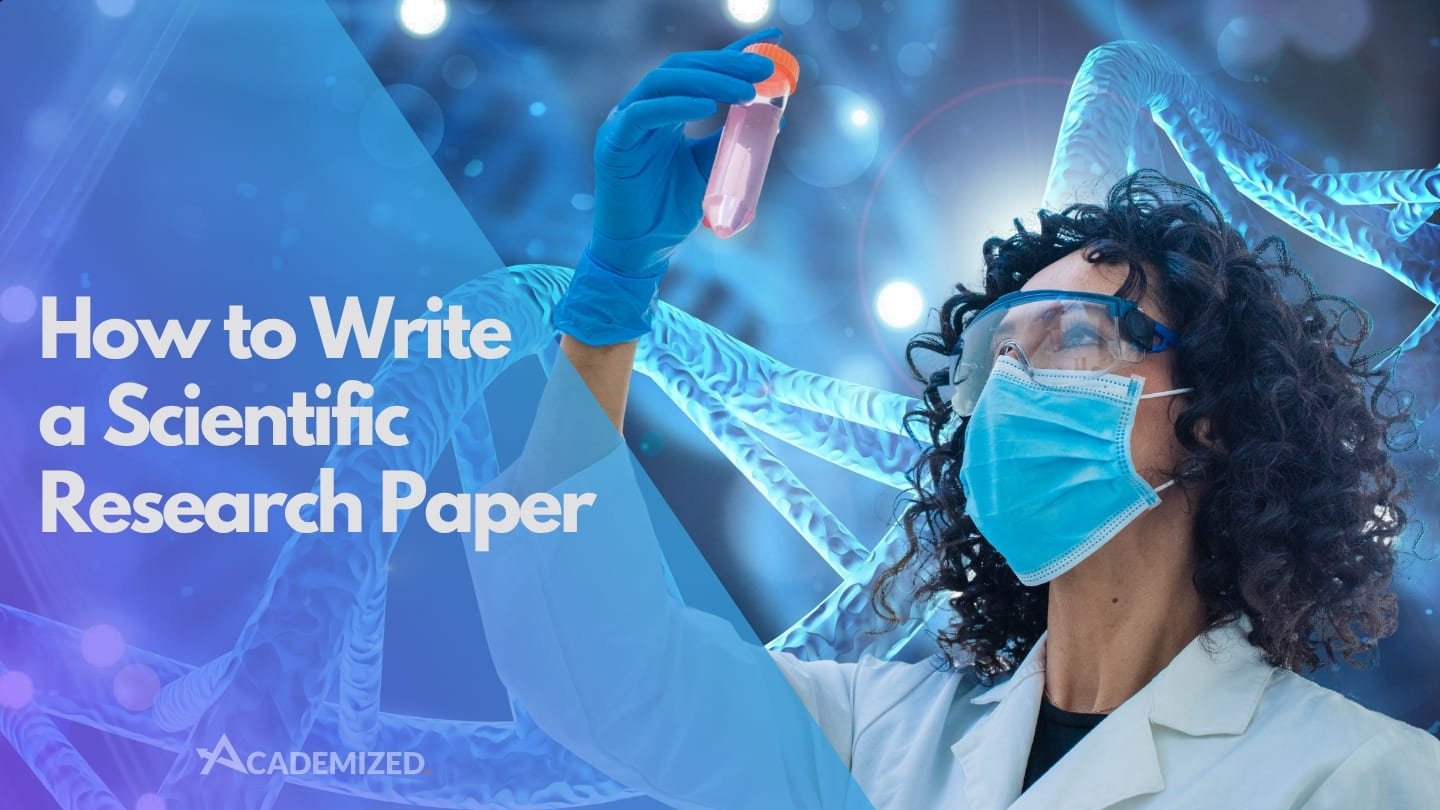
Research Paper Guides
by
Mary Watson
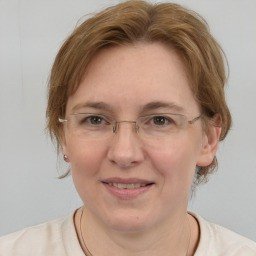
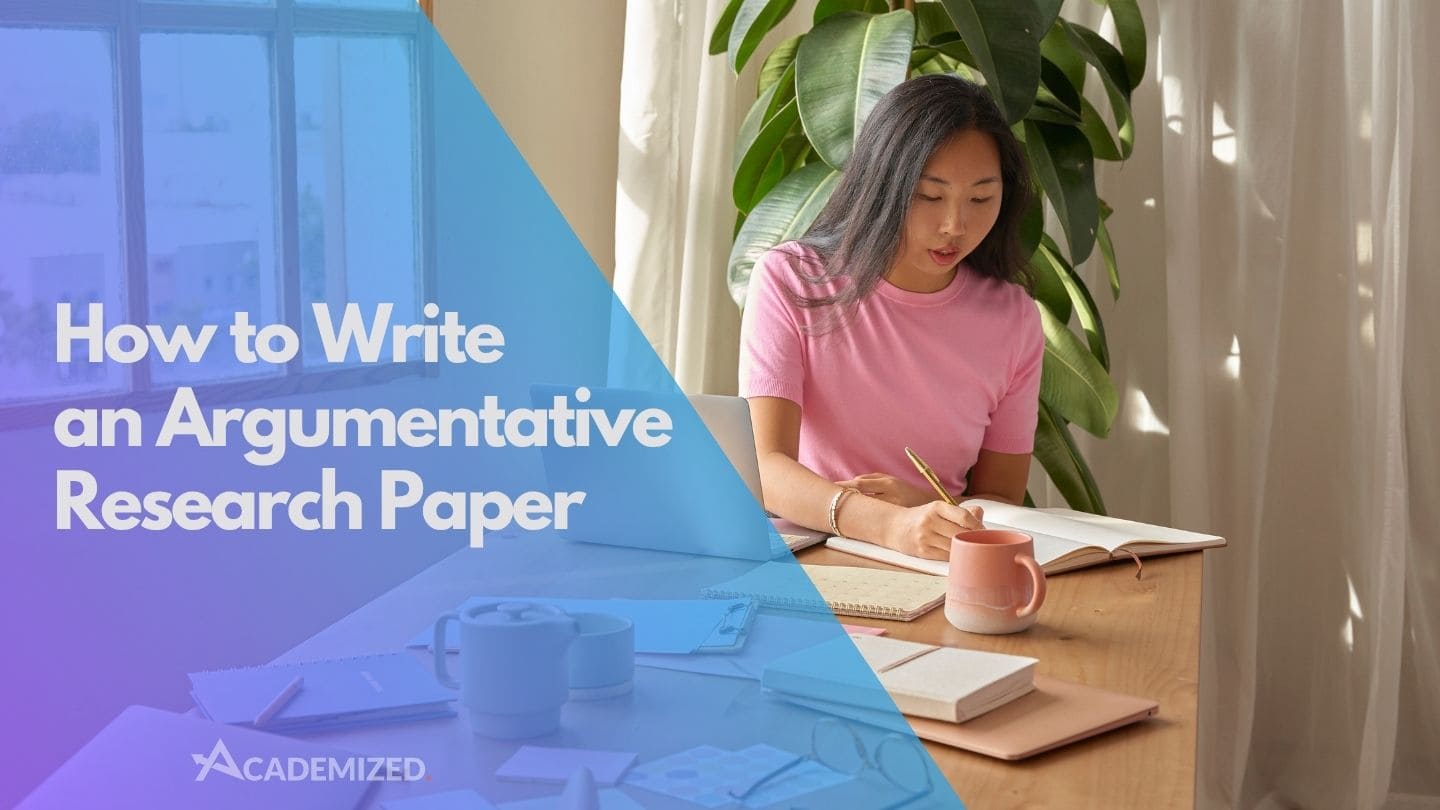